By Andrew Binder
In July of 1966, the New York Times ran a headline: “Mass of Ice as Big as Manhattan Moving Down Valley in Yukon: A Huge Glacier on Mt. Steele Has Torn Loose and Is Surging 2 Feet an Hour.”
In 2016, reporter Robinson Meyer likened the Kolka Glacier on the Russia-Georgia border to “the icy equivalent of a pyroclastic flow of hot gas and rock that gushed out of Vesuvius and flattened Pompeii.”
Today, the phenomenon of fast-moving glacier surges still perplexes scientists and continues to threaten human settlements.
The vast majority of glaciers sloth down mountainsides steadily, moving inches per day. But a subset of all glaciers – about 2,300 or 1 percent worldwide – exhibit strange behavior, oscillating between periods of slow and fast flow.
These icy behemoths known as surge-type glaciers inch forward for several years to centuries and then abruptly surge to 5-100 times the typical speed, sometimes moving 400 feet per day. After 1-5 years of fast flow, the glaciers decelerate to a normal pace. Although ink has been spilled on this topic for more than 100 years, predicting the surge – the switch from slow to fast – remains a mystery.
New research from scientists at the Massachusetts Institute of Technology (MIT) and Dartmouth College offers clues to understanding the trigger mechanics behind glacial surges. The work pioneers a new line of inquiry that may improve accuracy for early alarm systems that could protect vulnerable populations.
The physics-based model was published June 3 in The Royal Society Publishing, Proceedings A.
Forecasting a natural disaster
Scientists couple field observations with computer simulations to forecast earthquakes, volcanic eruptions, landslides, forest fires, floods and tsunamis. Similarly, accurate forecasting of surge-type glaciers requires understanding the environmental conditions that coincide to set the surge in motion – squeezing a trigger that initiates the event.
Co-authors Brent Minchew and Colin Meyer adapted earthquake and landslide mechanics to glaciers to create their model, which is one of the first physics-based simulations to explain the phenomenon of surges.
According to Meyer, the first key property is the surface beneath the bottom of the glacier. Observations of surge-type glaciers reveal that the glacier’s bottom surface slides over a bed composed of unconsolidated glacial till – a spongy mixture of boulders, gravel, sand and mud.
Yet, “nearly all of the theoretical work to date on glacier surging has adopted a hard- bedded model, meaning ice overrides impermeable bedrock,” said Gwenn Flowers, a glaciologist at Simon Fraser University who was not affiliated with the study.
Minchew and Meyer’s model reconciles this discrepancy between theory and observation. Describing the hard-bedded model, Meyer said, “this idea has a lot of intellectual inertia behind it but not that much actual data supporting it.”
The second key property is the glacier’s highest-altitude area, which is called the reservoir zone because it accumulates the most snow. The reservoir zone thickens over time until a downstream mass transfer occurs, and the glacier stretches downhill onto the valley floor – much like an inchworm stretching forward.
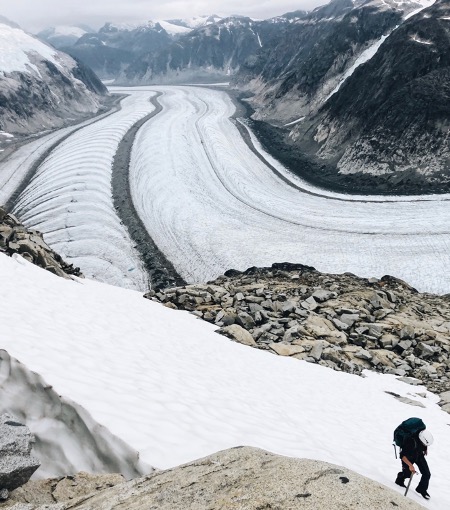
As mass moves downhill, the reservoir zone thins, and the glacier’s weight decreases. Picture a plate weighing down a sponge on a wet surface – when you lift the plate, the sponge expands and absorbs water. Like the plate, the glacier reduces its load on the spongy till below, freeing up pockets that fill with water and weaken the bed.
Over enough time, the conditions coincide – squeezing the trigger that initiates the surge – and the water-saturated till buoys the glacier up, reducing friction and initiating a rapid slide downhill.
“Most valuable, in my view, is the testable hypotheses that the paper produces,” said Flowers, “for example, the sharp boundaries that delineate surging and non-surging conditions.”
The development of a surge depends on key properties related to the rates of till weakening and mass transfer. Minchew and Meyer identified the critical ranges for both processes and suggested the environmental conditions that give rise to the key properties.
Developing an early response strategy
This June, The Green Climate Fund launched a $37 million initiative to build early response strategies in northern Pakistan. New equipment – including flowrate and depth meters, and drone and satellite imaging – will help authorities detect glacial surges with enough time to safely evacuate at-risk settlements.
The capacity to predict, and not only detect, glacial surges will require further advancement of Minchew and Meyer’s model. For now, the physics-based framework opens a path to future discoveries that could explain one of Earth’s most terrifying icy phenomena.
Andrew Binder is a senior at Dartmouth College majoring in earth sciences with a minor in creative writing. He is an editorial intern at Providence Media and a former copywriter for GoPeer, an educational technology company. He is the science editor for his school’s independent student literary magazine, Meetinghouse. Follow him on Twitter @andy_rednib or email him at andrewebinder@gmail.com.
This story was produced as part of NASW's David Perlman Summer Mentoring Program, which was launched in 2020 by our Education Committee. Binder was mentored by Danielle Torrent Tucker.