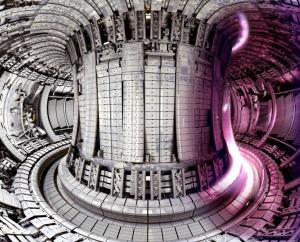
The inside of the Joint European Torus (JET). The virtual overlay on the right shows the plasma (pink, its authentic color) within the magnetic field, hovering just a few inches from the armored walls of the reactor. Credit: EUROfusion Consortium
WASHINGTON — Scientists worldwide are working toward clean energy, but current approaches are among the most complex ever attempted. To keep pressing forward, researchers have looted the toolbox of high-energy physics for new ways to measure phenomena under extreme conditions.
One of the most alluring but ever-distant approaches is controlled nuclear fusion — the reactions that power stars. Precision is paramount for fusion reactors to succeed, speakers said during a Feb. 14 symposium at the American Association for the Advancement of Science (AAAS) annual meeting.
“Measurement really is the fuel of progress,” said physicist Joanne Flanagan of the Culham Centre for Fusion Energy in Abingdon, U.K. “You really need to know that your ‘whizzy science machine’ does what your theory says it will.”
These “whizzy machines” in the quest for clean energy range from ultrathin solar cells to catalysts that split water molecules. High-energy lasers and synchrotron radiation — a powerful form of X-rays generated by particle accelerators — allow physicists to peer into the atomic structures and behaviors of these novel materials.
Flanagan and her colleagues at the Culham Centre work on a cleaner nuclear energy with a particularly flashy machine: a donut-shaped magnetic field with the Russian-derived name tokamak. In the minute-long bursts during which it runs, their test device, called the Joint European Torus, or JET, contains a tiny, man-made star.
Unlike traditional nuclear energy, which depends on an atom-splitting process called fission, controlled fusion can produce large amounts of energy with no threat of a runaway reaction.
Flanagan and her team heat hydrogen atoms in the tokamak to 150 million degrees Celsius — 10 times the temperature at the sun’s core. Groups of four superheated nuclei fuse to form helium atom nuclei, a final product with slightly less mass. The lost mass is converted to energy.
At such high temperatures, it is difficult to measure what happens inside the tokamak. Instruments and even the reactor walls would melt on contact. A strong magnetic field keeps the hot gas, called plasma, in the shape of a hovering donut, a few inches away from the reactor walls.
Flanagan monitors temperature remotely by shining an infrared laser into the tokamak. The plasma scatters light back like a prism. The broader the spectrum, the hotter the plasma.
JET bristles with more than one hundred measuring instruments.
“The reason for that variety is because JET is a research box,” Flanagan said. “Its reason for existing is to produce data for the scientists to do research.”
This research will help inform aspects of the construction of a larger tokamak, called ITER, being built in France. Another tokamak, DEMO, will follow. Physicists hope DEMO will demonstrate that fusion is a viable option for future energy needs.
Traditional nuclear power accounts for about 20 percent of the energy produced in the U.K. and the U.S. However, storing radioactive waste from the process is a delicate business. Sending it into space, the ocean or a subduction zone risks an unexpected resurfacing in centuries or millennia.
“We haven’t got many options,” said Claire Corkhill of the University of Sheffield, U.K., at the symposium. The “least-worst option,” she said, “is geologic disposal.”
To confirm whether engineers can safely store nuclear waste in cement containers deep underground, Corkhill must examine tiny cement particles at a microscopic level and over geological timescales. The basic question: How would this cement hold up over time?
Modern nuclear waste will take between 100,000 and one million years to decay to safe radiation levels. “We’re looking at civilizational timescales,” Corkhill said.
She investigates the bonding properties of different recipes of cement to determine which might best trap radioactive waste over millennia. When cement begins to set, it undergoes rapid reactions. After it solidifies, it continues to change over many years. This combination makes its properties difficult to measure.
Corkhill is working with synchrotron X-rays from the U.K.’s Diamond Light Source to investigate the reaction stages or “phases” of cement in a sample over the course of two years. The results will inform a long-term model. A new experimental probe with the X-rays has uncovered traces of unknown phases in the cement, Corkhill said.
Like Flanagan, she hopes these new discoveries will make clean, safe technology practical on an industrywide — and ultimately global — scale.
Elizabeth Jacobsen is a senior at Williams College, studying biology and English. She writes for The ScientEphic:Williams College Science Blog, and has written for The Plant Press, a publication of the Smithsonian National Museum of Natural History. Reach her at Elizabeth.Jacobsen@williams.edu.